we had briefly seen the fundamentals of ice covers a ship may encounter. We had also touched upon the concepts of statistics, mechanics of ice load. Now moving on we venture into the design peculiarities of these specialised vessels and their features, capabilities and classification.
We had differentiated between Ice Class vessels and Icebreakers. Here looking into this aspect, there are mainly 3 breeds of ships which we need to contemplate upon:
Ice-Strengthened Ships: These come under ‘Ice-Class Vessels’ and have additional ‘ice-strengthening’ and ice capable performance which qualify it to move in icy waters. Again by ice-capable performance, we mean adequate engine power rating, specialised propulsion, machinery, high-end material provisions in areas of higher vulnerability etc. They require an escort of icebreakers while moving in ice as they are not self-sufficient to break or plough through ice especially in more drastic conditions. They do not have significantly altered hull design and are characterised by a common bulb form bow (bulbous bow).
Ice-going vessels: They are a higher breed of ice-strengthened ships not having the requirement on an icebreaker escort. Their hull form, build, propulsion, navigational features and most importantly, strength are incorporated to exclusively function in icy waters.
Icebreakers: They are specialised vessels with the sole purpose of breaking through all kinds of ice conditions and escort other vessels as discussed above. Goes without mentioning that they have all high-end features of ‘Ice-Class’. An icebreaker basically makes a channel after breaking through the ice cover. This makes way for other vessels to move by. It has been observed that the width of the made channel is roughly two ice thicknesses more than the ship’s beam.
All three of these ships have varied similarities and differences. However, the principal purpose of all of them remains the same in varying degrees: to negotiate and sustain heavy moduli of ice loads without affecting the structural strength and functionality of the vessel during operation.
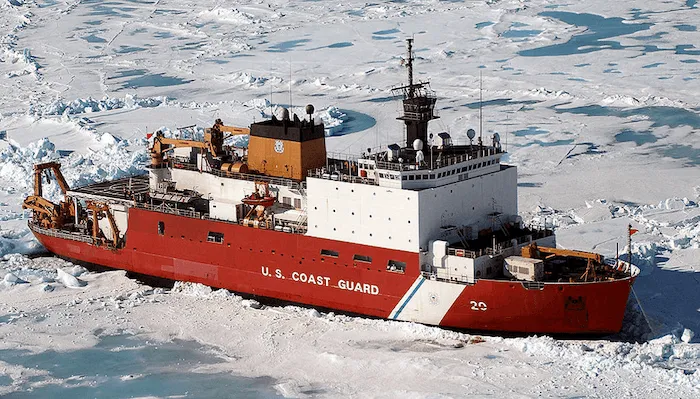
Image 2: Icebreaker USCGC of U.S Coast Guard
From the hydrodynamic analogy, the ice loads too can be segregated to:
· Global Loads
· Local Loads
Local loads can be described as loads coming from single site ice contacts at multiple locations or a fraction of a larger loading on a particular hull element. For instance, if we have a cusp of ice contacting a part of a hull, then it’s a local load! Or if we divide a larger contact force acting on a particular plate panel on a certain frame during a certain span of time, then that’s again a local load. This can be depicted in the figure below.
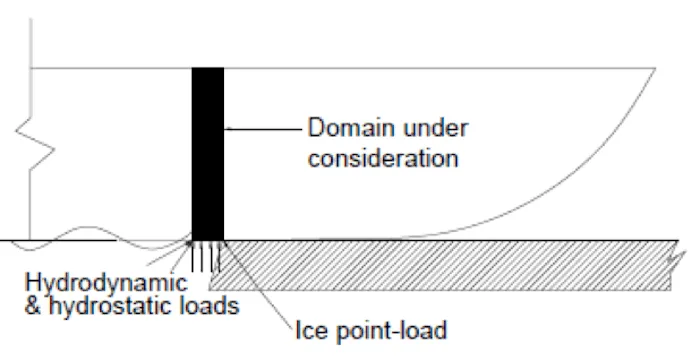
Figure 3: Depiction of localised ice loads acting on a ship
On the other hand, the total load from an ice cover acting on the larger elements like the entire hull girder or say, the side shell plating longitudinally is the Global Loading. To put it simply, the sum total of various forces simultaneously acting on the entire ship is the global load.
This is the most crucial aspect for a designer in terms of selecting the hull form, structural characteristics, build specifications and the build material. Like hydrodynamics, global ice loads consider the hull girder as a single simply supported beam enacted upon by distributed loading from ice and water.
Basically, the load response on the hull is time-variant reaction forces which occur due to various load incidence processes.
These loads are:
· Forces due to crushing/breaking the ice
· Forces due to breaking by bending or flexural failure (buckling)
· Forces by submergence of ice
· Frictional forces due to sliding, grazing or breaking of ice fragments along the hull (Sliding Forces)
· Open water hydrodynamic forces
These above are nothing but different failure modes of ice. Ice exhibits varying strength against these different failure modes. These somewhat depend on the nature of ice and impact area. Brash or fragment ice has lesser strength. Also, owing to their physical size, the area of impact is small. Thus they are easily yielded by crushing. Consequently, the impact pressures upon the vessel are less. Breaking or crushing of ice begins when the stem of the ship comes in direct contact with the ice cover.
The ice gets grinded into smaller fragments which get dispensed in the surrounding water. This continues for some time. Then at a certain distance away from the point of collision, the impact forces are transmitted. A similar situation can be visualized. Suppose you pelt a piece of stone onto a glass anywhere. At the point of impact and the vicinal areas, the effect is more to form a cavity.
However, slowly tracing the fault, cracks can be observed up to longer extents varying from intense ones to fine hairline ones directly depending on the distance from the point of impact. Now if this sheet is subjected to some continuous force or even tampered more, there is a high possibility of a shear failure at some distance away from the impact and perhaps a consequent breakage. So happens in case of ice sheets. The increasing distance from the point of impact creates linearly increasing bending moment that leads to gross shear failure after a certain point. Obviously, this is the case mostly for more expansive ice covers or bergs whereas smaller fragments are mainly yielded by crushing.
Often the vessel may climb over an ice sheet during the interaction process. As a result, the bending failure happens partially owing to the vessel’s weight. This leads to submergence loads.
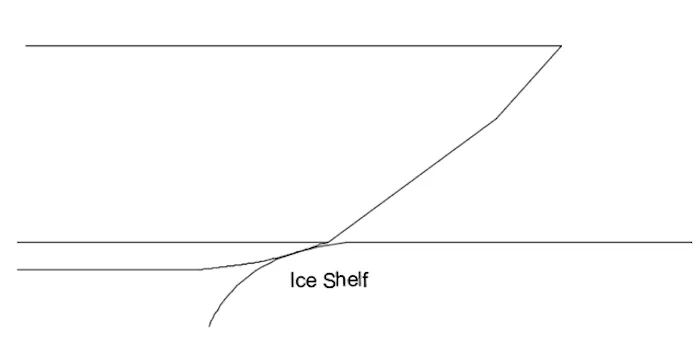
Figure 4: A vessel tending to climb on ice
Friction is a ubiquitous aspect. Ice is definitely not an exception. The grazing, rubbing, collisions and breakage of ice pieces in various degrees create significant modulus of frictional forces. These enact locally but aggregate to give rise to resistance forces affecting the overall surge. Not to forget the ‘localized’ impacts on the hull plating due to their continuous interactions. Also, last but not least, open water hydrodynamic loads that are always persistent.
Friction from ice is an integral part of ice resistance about which we shall delve at a later course. But at this stage, it suffices to state that the surface properties of ice affect the ‘abrasive’ properties, which in turn impact the friction forces enacted upon by the incident ice and consequently, Ice Resistance.
Now obvious physical properties of ice come into play like the strength of the ice, thickness and density. These basic physical properties dictate the nature of ice, which in turn affects ice loads and consequently, resistance.
Now here is an interesting thought to ponder upon at this point. From a structural point of view in case designing ships, buildings or any other civil structures, we dedicate our heart and soul in fulfilling its minimum parameters such that it endures loads without FAILING. All physical parameters are looked upon such that they are good enough to sustain forces. This is exactly reverse in case of ice! We dedicate our heart and soul in overpowering its physical values such that they fail easily without damaging the vessel or jeopardising its operability. Also, minimising encountered Ice Resistance.
Before discussing resistance, let us look at how these physical properties affect ice behaviour and their induced loads.
Ice Thickness
Ice thickness, as seemingly obvious in determining the strength and thus the ice loading. This thickness, is in turn, determined by a number of factors such as freezing time, ambient temperature, snow type, wind speed etc. More the freezing time, lesser the ambient temperature, lesser the wind speed (due to its wearing effect), the thicker the ice grows. This is, of course, related to the age of ice, as discussed in our last article.
Density
With the word density, the foremost thing that comes to our mind is weight coupled with compactness. This influences the impact force and also the buoyancy. The buoyancy of icy waters has been a complex phenomenon. Apparently, as the buoyant force is provided by water itself, the role of ice is often neglected. However, as ice is much denser than water, a smaller fraction of icy water can provide the required buoyancy force equivalent to a larger volume of full water. Also, in instances when the vessel climbs up a sheet of ice, the lift forces accounted for is quite significant. This is interrelated with two other very significant parameters, salinity and temperature.
Salinity is the ratio of the salt content in ice versus the mass of ice. This increases the density as expected. Another factor comes into play, namely the temperature. Observations reveal that with increasing salinity, density is more sensitive to temperature changes. The physics is simple. Higher salinity implies more brine content, more sensitive to temperature changes.
Porosity
All ice have an inherent porosity characteristic. This is tantamount to the amount of gas trapped inside and brine content, affecting its rigidity and strength. This is directly proportional to the salinity and inversely to temperature for obvious reasons. Furthermore, the size and distribution of the pores also affect the resultant strength of the ice cover under consideration.
Flexural Strength
Probably it is one of the most crucial physical aspects of ice. Flexural strength is the measure of the ice’s ability to bend before failing. As the name suggests, flexural strength is the inherent material property, conceived as the maximum permissible stress sustainable by the material before it ruptures due to bending loads. This flexural strength is in turn, directly proportional to brine fraction. So, ice in more salty waters tends to be stronger!
Breaking Process of Ice
Now the main breaking process of ice is an interplay of these various physical properties in the incidence of the stem of the vessel ramming through an expanse of ice. The most commonly encountered ice condition is ‘level ice’. Though other several ice forms exist like ridges, floes or pack ice, the most convenient way to depict the ice-breaking process is through level ice. This can be very well illustrated in the following figure:
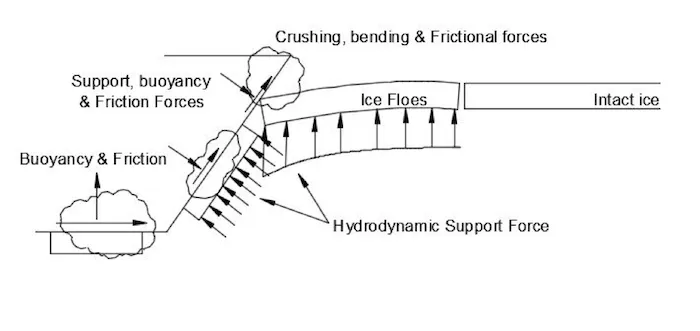
Figure 5: A typical ship-ice interaction force distribution
In the initial stages, when the ice sheet is being impacted upon, numerous local loads are generated in the stem due to the ‘crushing of ice’. Small fragments of ice in the form of cusps and floes are generated. This process continues for some time. Gradually, the ice sheet is being delivered upon by a longitudinal force in terms of flexural loading, in turn, caused by compression and buckling loads. Tantamount to this is the submergence load in case of the vessel tending to climb upon the ice. This leads to huge bending and buckling loads on to the ice sheet gradually affecting its flexural strength.
As the crushing/ breaking action is already in an advanced stage by this, time the surface area increases for the action of the contact force. This increases the vertical component of the contact force of ship-ice interaction at the bow from the simple relation of Force= Pressure* Area, the pressure being from the enacting ice load.
This coupled with the ‘climbing force’ of the vessel leads to the formation of a bending crack. After a certain point of time, the ice sheet breaks off at the point of maximum bending load. The other forces acting during this interaction process are local reaction forces (at the point of contact during breaking), friction forces from the grazed off ice floes, hydrodynamic forces and of course, the support and buoyancy forces.
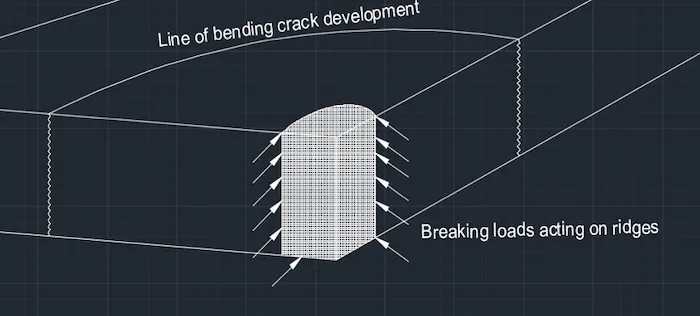
Figure 6: A visualisation of ice-breakage leading to bending
After the breakage, the ice gets disintegrated to floes, cusps, bergs or other smaller fragments. Now, these entities of ice pose a different kind of problem in terms of ice loading, resistance and hydrodynamics. Though these are a bit beyond the scope of this article, in the next part we will try to brush up on their effects in a very concise and simple way.
Comments are closed