Estuaries and continental shelf areas comprise 5.2% of the earth’s surface and 2% of the oceans volume. However, they carry a disproportionate human load [14, 51]. Coastal and estuarine waters receive contaminants via local anthropogenic activities and riverine inputs [9]. Chemicals that are released into aquatic systems are generally bound to particulate matter, which eventually settles and becomes a part of the water-sediment system. Therefore, aquatic sediments are the ultimate sink for many pollutants including metals. Toxicity and other adverse effects in sediments are functions of the total concentration and bioavailability of the sediment-associated chemicals. Specific metal forms are useful environmental indicators because the fractionation of the total metal contents may indicate their origin [12, 13, 42].Currently, numerous natural and anthropogenic hazards expose the tropic aquatic environments to multiple pressures [2, 47]. The Cai River and the Nha Trang Bay form one of the major estuarine systems in the South China Sea, which is inhabited by unique biota. This area, which was wilderness not long ago, now experiences a significant anthropogenic load from the local people activities and particularly the quickly growing touristic industry. Moreover, aquaculture, which is currently one of the most rapidly developing production sectors in coastal Vietnam, contributes to the degradation of estuarine and coastal ecosystems. In the recent decades, significant research efforts have been undertaken to evaluate the biodiversity and ecosystem community structure in the Nha Trang Bay [5, 36-38].
However, notably few data are available relative to the contamination levels and trends. The mercury concentrations in the riverine, estuarine and marine sediments of the region were reported to be at or below the natural levels [24, 25]. The aquaculture activity has been reported to contribute to the ecosystem with heavy metals in the farming environment in Nha Trang Bay and Van Phong Bay [35]. Elevated metal concentrations were obtained in macrophytes that were collected in estuarine waters in the city of Nha Trang [8]. The study on the sediments from coastal lagoons of Khanh Hoa Province reported generally low metal levels that were derived from rocks and soils of the watersheds [42].The first geochemical study of the Nha Trang Bay covered the distribution of TOC, n-alkanes and the bulk concentration of major and trace elements in the surface sediments [1, 17, 25, 38]. Further analysis and speciation of metals in the sediments of the Cai River estuary and Nha Trang Bay will allow one to track the fate of potential contaminants. Therefore, the objective of the present study is to summarize the data on the abundance, distribution and bioavailability of major and trace elements in the surface sediments of the Cai River estuary under multiple stresses. Because the chemical form or speciation is the most important factor that influences bioavailability, the specific goal of this study is a comparative analysis of the ecologically significant chemical forms of metals.
Material and methods
ENVIRONMENTAL SETTING
The Cai River, its estuary and the adjacent part of the Nha Trang Bay belong to the Central Southern Coastal Region of Vietnam (Khanh Hoa Province) (Figure 1). The regional climate is monsoonal subequatorial with a yearly average temperature of 23–24°C. The rainy season lasts from September to December or January. Rainfall reaches 1,300 to 1,400 mm/year. In the last decades, climate change events such as anomalous precipitations and droughts were observed in the region [37].The relief is mountain-hilly. The main soils of the region are yellow- and red-coloured ferralitic soils on acid and alkaline magmatic (mainly basalts and andesite-basalts) rocks, whereas the river deltas are covered with alluvial soils [34, 36]. The natural terrain is mainly covered with broad-leaved, mixed-leaved and mangrove forests. The soils of the agricultural terrain are used f or crops of rice, maize, sweet potato and cassava.
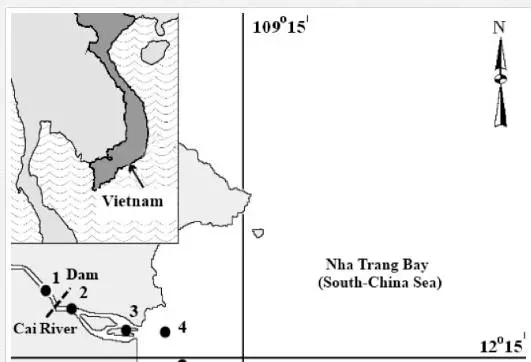
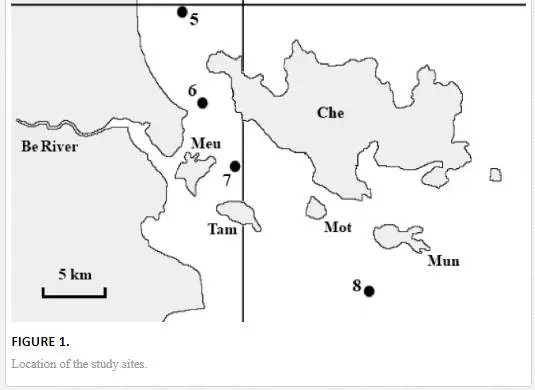
The length of the Cai River is 80 km, its catchment area is 1,450 km2, and the annual river discharge is approximately 2 km3. The fresh-water discharge of the Cai River is highly seasonal and varies from 10–15 m3/s in the dry season to 600–700 m3/s in the rainy season. The main river discharge is deflected to the South coast of the Nha Trang Bay along the ancient riverbed (channel) [36]. The fresh river water (S< 0.1‰) and saline South China Sea water (S ≈ 36‰) form a major water-mixing zone. The fill dam that was constructed in 1998–1999 eight kilometres upstream from the river mouth restricts the water exchange and marks the riverine boundary of the water-mixing zone (Figure 1). The Cai River estuary and Nha Trang Bay can be divided into three sub-zones: I – river (S < 0.1‰), II – transitional waters (estuary) (0.1‰ > S > 32‰) and III – sea (bay) (S > 32‰). In the transitional waters, the salinity (S) increases from the river to the sea and from the surface to the bottom. The water column is highly stratified with the pronounced horizontal and vertical salinity gradients [25, 36]. The hydrological regime of the estuarine zone of the Cai River is complicated by daily tides (the tidal range reaches 2 m), seasonal fluctuations in the fresh-water discharge and monsoonal seawater upwelling. The daily tides lead to regular upstream invasions of the near-bottom saline water lenses, which are limited by the dam. The seasonal variability in the Cai River discharge significantly influences the estuarine zonation. Thus, in the rainy season, the fresh water flows seawards beyond the Che Island (Figure 1), whereas most river sediment is carried far to the Nha Trang Bay with the river water in the upper layer [36].
The major part of the Cai River estuary is located inside the Nha Trang city (300,000 inhabitants, 100,000 tourists in the season). The estuarine water flows through the residential and industrial zones with their ports. The outflow of waste and sewage sludge and the shipping, fuelling and vessel production and repair are sources of the local anthropogenic contamination of the estuary. The estuarine and coastal zones of the Nha Trang Bay are a farming environment for aquaculture, where green mussels, babylonia snails, abalone, sea cucumber, red snapper, sea bass and lobster are cultivated. The agrochemicals and veterinary drugs that are widely used in aquaculture production may be an additional source of anthropogenic heavy metals in the Nha Trang Bay area [35].
The climate variability (seasonality and precipitation), extreme weather events (droughts, storms and upwelling) and human activities (deforestation, urbanisation, land use, damming, tourism, coastal construction, transportation and fisheries) expose the Nha Trang Bay to multiple pressures that contribute to further degradation of the estuarine and coastal ecosystems in the region [14].
FIELD WORK
The water and sediment samples were collected in the Cai River estuary and Nha Trang Bay on 6–10 July 2010 at eight locations along the salinity gradient. The sampling stations were located in the riverine (st. 1), transitional (sts. 2–4) and marine (sts. 5–8) sub-zones of the dry season (Figure 1).
The surface and bottom water samples were obtained using a plastic Niskin bottle. The temperature, alkalinity and salinity of the water samples were measured on board immediately after collection using portable conductivity apparatuses HI 98129 Combo and HI 98302 DIST 2 (Hanna Instruments, Germany). The surface sediment samples were obtained by scuba-divers with a manual plastic piston corer, which was designed at the P.P. Shirshov Institute of Oceanology (Russia). Three samples were collected at each location. On board, the visual estimates of grain size and the colour and relative proportion of the components were made and recorded [30]. The upper layer (0–6 cm) of the samples was retrieved using stainless steel spatulas. Then three upper layer samples were mixed into a one composite sample. The composite sample was transferred into pre-cleaned polyethylene containers and frozen until arrival in the laboratory. One portion of the composite sample was kept frozen until the grain size and mineralogy analyses. One portion was dried to constant weight at 60°C until the major-, trace-element, TOC and TIC analyses. One portion was dried to constant weight at 40°C until the Hg analysis. The sampling, sampling transportation and preparation procedures were performed using standard clean techniques that were described elsewhere [25, 26, 30].
ANALYTICAL METHODS
Three samples representative of the surface sediment at the riverine (st. 1), transitional (st. 4) and marine (st. 8) locations (Figure 1) were subjected to extensive grain size and mineral composition analyses. The grain size analysis was performed by wet sieving. The mineral composition of the total samples was determined on the X-ray diffractometer D8 ADVANCE (Bruker AXC, Canada) [31].The total Si content in the samples was determined using the X-ray-fluorescence method on the SPECTROSCAN MAKC-GV WDXRF spectrometer (Spectron, Russia). The total carbon (TC) contents in the samples were determined by dry burning at 900ºС in oxygen flow and the total inorganic carbon (TIC) contents were determined by dry burning at 200ºС with H3PO4 with the analyser TOC 5000-V-CPH (Shimudzu Co., Japan). The total organic carbon (TOC) contents were determined as a difference between TC and TIC contents in the samples [38]. For the total Al, Fe, Ti, Mg, Ca, Na, K, S, P, Mn, Li, V, Cr, Co, Ni, Cu, Zn, As, Sr, Zr, Mo, Cd, Ag, Sn, Sb, Cs, Ba, Pb, Bi and U content analysis, the samples were subjected to the total acidic dissolution in HNO3+HF+HClO4 in an open system with further determination of element contents using the ICP-AES and ICP-MS methods (Thermo Scientific, USA). The detailed sample decomposition and analytical procedures are described elsewhere [15]. The Hg content was determined in the dry samples using a pyrolyse method on the RA-915+ spectrometer with background correction and a two-chamber atomiser PYRO-915+ (Lumex, Russia) [25].
To assess the chemical form of selected metals (Fe, Mn, Li, Cr, Zn, Cu, Pb, Ni and Ag) in the sediments, the samples (excluding sediments with the highest sand (st. 5) and carbonate (st. 7) content) were subjected to single chemical reagent (single-step) extraction procedures. The strong-acid-soluble metals were extracted using 2 M nitric acid, weak-acid-soluble (labile) metals were extracted using 25% acetic acid, dithionite-soluble metals were extracted using sodium dithionite at pH 6.5–7 (Mehra-Jackson extraction) and oxalate-soluble metals were extracted using ammonium oxalate–oxalic acid buffered solution at pH 3.2 (Tamm extraction). To isolate the weak- and strong-acid-soluble metals, 15 ml of 25% acetic acid and 15 ml 2 M nitric acid, respectively, were added to 1.1 g of dry sample in polypropylene vials and shaken in a mechanical shaker for 6 h with acetic acid and 1 h with nitric acid. Then, each extract with the sediment was filtrated into a 25 ml glass volumetric flask. The sediment on the filter was washed with 10 ml of distilled water, and the wash water was added to the flask [30]. To isolate the non-silicate iron compounds and the microelements that are associated with this phase, 20 ml of 0.3 M sodium citrate and 2.5 ml of 1 M sodium hydrocarbonate were added to 1.1 g of dry sample in a centrifuge vial and heated on a water bath up to 80°C; 0.5 g of dry powder sodium dithionite was added, shaken and heated for 15 min at 80–85°C; then, 5 ml of fat NaCl solution was added and centrifuged for 10 min at 3,000 t/min. Then, the extract with the sediment was filtrated into a 250 ml glass volumetric flask. The sediment was subsequently subjected to two more repeated extractions, and the extracts were added to the 250 ml volumetric flask [50]. To isolate the amorphous iron oxides and their associated microelements, 50 ml of ammonium oxalate–oxalic acid buffered (Tamm) solution was added to 1.1 g of dry sample in the 250 ml flat bottom flask, shaken for 1 h and filtrated to a 250 ml glass volumetric flask. The sediment on the filter was washed with 10 ml of distilled water that was mixed with a small amount of oxalic acid, and the wash water was added to the flask. Then, the filter with sediment was added to the sediment in the flat-bottom flask and subjected to one more repeated extraction, and the extract was added to the 250 ml volumetric flask [49]. The metal contents in the extracts were further determined using an atomic absorption spectrometer (AAS) Hitachi 180-8 (Hitachi Co., Japan) in the Analytical Centre of Moscow State Lomonosov University.The relative accuracy of the analytical determinations was within the standard deviations that were established by the certified reference materials (CRM) SDO-1 (Russia) (for SiO2, TOC and TIC), SRM 521-84Р (Russia) (for Na2O, Al2O3, Stotal, K2O, CaO, MnO, Fe2O3, Li, V, Cr, Zn, As, Sr, Zr, Mo, Ag, Sn, Ba, Pb), AGV-2 (USA) (for MgO, P2O5, TiO2, Co, Ni, Cu, Sr, Sb, Cs, U) and Mess-3 (Canada) (for Cd and Hg).
Results
WATER COLUMN CHARACTERISTICS
In July 2010, the salinity of the surface fresh-water layer along the river–sea (sts. 1–8) transect varied from 0 to 35‰, and the salinity in the near-bottom water layer varied from 0 to 36‰ (Table 1). The frontal zone of the contact of fresh and saline waters occurred downstream from the fill dam (sts. 2–3, Figure 1), where the vertical salinity gradient was 2.3–3.7‰ per 1 m depth, and the horizontal salinity gradient was 1.5–9‰ per 1 km distance. The temperature (T) of the water column varied in narrow ranges and decreased from the river to the sea from 30°C to 29°C in the surface water layer and from 29°C to 26°C in the near-bottom water layer. The pH of the water column increased from neutral in the riverine waters (pH 7, st. 1) to low-alkaline in the transitional and sea waters (pH 8–9, sts. 2–8).
TEXTURAL AND MINERAL COMPOSITION OF SURFACE SEDIMENTS
The on-board textural estimates indicate that the surface sediments of the studied part of the Cai River–Nha Trang Bay estuarine system mainly consist of brownish sandy mud (sts. 1–5) and fine-grained greyish mud (sts. 6–8). The oxidised region (one to three or four centimetres of sediment) is typically orange–brown because of Fe and Mn oxide accumulation. Resuspension of the surface sediment layer is observed in most of the transitional and marine locations (sts. 2–6).
The percent of the content of sand- (63 µm–2 mm), silt- (2–63 µm) and clay- (<2 µm) sized material and the mineral composition were determined in three sediment samples, which were collected at the riverine (st. 1), transitional (st. 4) and marine (st. 8) locations (Table 1). The coarsest sediment is from riverine station 1. Downstream in the transitional sub-zone, at station 4, the sediment contains less sand and more silt. The most fine-grained sediment, with the highest clay content, is from marine station 8. The surface sediments of the Cai River–Nha Trang Bay estuarine system mainly contain quartz (33–67% of the total dry weight), kaolinite (4–11%), illite (8–12%), albite (5–11%), gibbsite (3–7%) and clinochlore (2–5%), which are present in each of the three studied samples. Microcline (5–8%) and aragonite (1–3%) are only present in the riverine and bay sediments (sts. 1 and 8). Hornblende (3–4%) and gypsum (1–2%) are only present in the estuarine and bay sediments (sts. 4 and 8). A significant content of orthoclase (11%) is only found in the estuarine sediment (st. 4). Calcite (5%) is only found in the bay (st. 8) (Table 1). By analysing the admixtures and individual mineral grains, traces of zircon, tremolite, ilmenite, sphalerite, pyrite, epidote, biotite, muscovite and the Ag- and Ag-Pb sulphosalts of the freieslelebenite and pyrargyrite groups were identified.
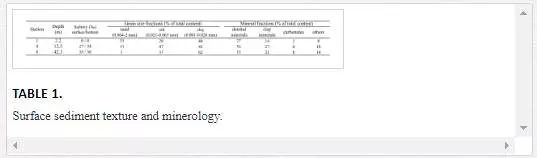
ABUNDANCE AND DISTRIBUTION OF MAJOR AND TRACE ELEMENTS IN SURFACE SEDIMENTS
The total contents of Si, Al, Fe, Ca, Mg, Na, K, Mn, Ti, P, S, TOC and TIC are reported in Table 2. The mean contents of the major elements are within the range of the Clark contents in shale, pelagic clays and average world riverbed sediments. However, the studied sediments are enriched with S and depleted in P in comparison to the reference values [22, 44]. Sedimentary Si varies in a narrow range and slightly decreases seaward along the river–sea transect. Sedimentary Al generally increases seaward and is elevated in the sediments from stations 2, 4 and 6. The distribution of Fe, Ti and Mg in the river–sea transect is similar to that of Al with a strong positive correlation (the Spearman rank order correlation coefficient (rs) ranges from 0.7 to 0.9 at p ≤ 0.05 and n = 8). Na, K, P and S show independent distributions along the salinity gradient in relation to the other studied elements but tend to increase seaward. The distribution of the inorganic carbon content in the sediments along the river–sea transect is characterised by a general increase seaward and a maximum in the sediment from station 5, which is rich in shell detritus. Sedimentary Ca is distributed similarly to inorganic carbon with the strongest positive correlation (rs = 0.98, p ≤ 0.05, n = 8). Sedimentary organic carbon (TOC) is uniformly low and exhibits no significant correlation with other elements that were studied.
The mean content of the major part of the studied trace elements (Li, V, Cr, Co, Ni, Cu, Zn, As, Sr, Zr, Mo, Cd, Sn, Sb, Cs, Ba, Hg and Pb) in the sediments from the Cai River estuary and Nha Trang Bay is lower or corresponds to the reference values for shale, pelagic clays and the average world riverbed sediments (Table 2). The exceptions are U, Bi and Ag. The mean content of U exceeds the reference values by 1.5–2 times; the mean content of Bi exceeds the reference values by 3–7 times; and the mean content of Ag exceeds the reference values by 40–80 times.
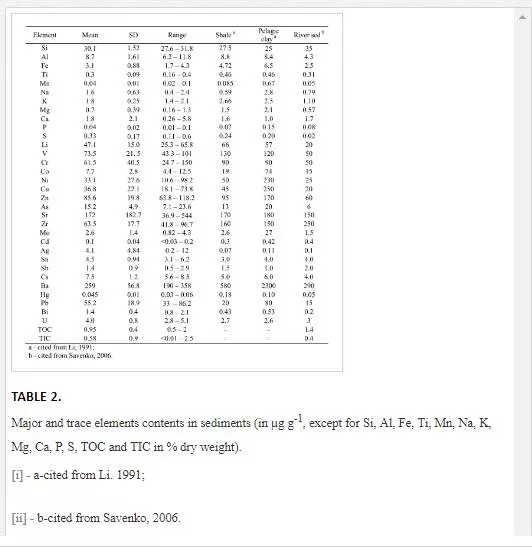
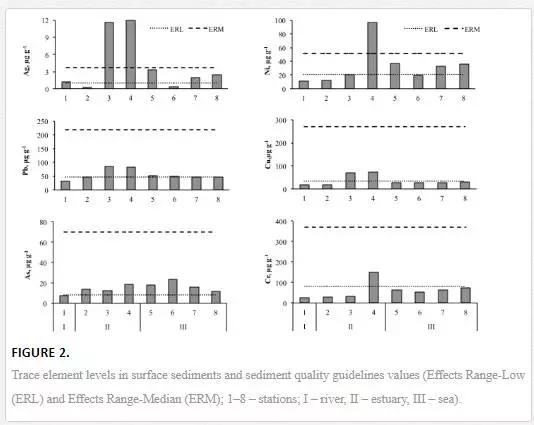
Figure 2 illustrates the trace element levels compared to the SQG (Effects Range-Low (ERL) and Effects Range-Median (ERM)) values along the Cai River–Nha Trang Bay transect (Long et al., 1995). The Ag content exceeds the ERL level in the sediments at most locations and is four times higher than the ERM value at stations 3 and 4. The Ni content corresponds to or exceeds the respective ERL value in the sediments at most transitional and marine locations (sts. 3–8) and is much higher than the ERM level in a single sediment sample at the transitional station 4. The Pb and Cu contents are much lower than the respective ERM values at all locations, and correspond to the respective ERL values at most locations while exceeding the ERL levels at stations 3 and 4. The As content is below the ERM level but exceeds the ERL value in the sediments from most transitional and marine locations. The Cr content is below the ERM level at all locations but exceeds the ERL level in a single sediment sample from station 4 in the transitional sub-zone. The Hg and Cd contents in the surface sediments at all locations are below the ERL and ERM levels.
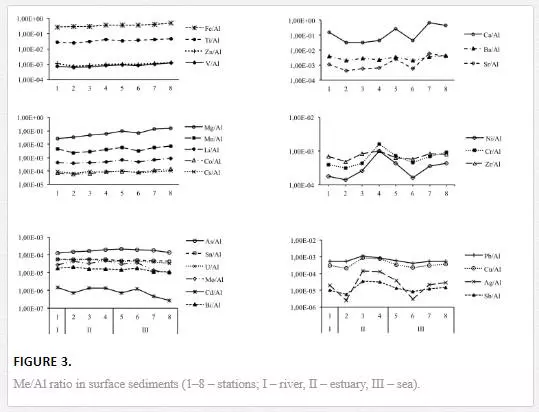
The distribution of metal/Al ratios along the Cai River–Nha Trang Bay transect is provided in Figure 3. The aluminium normalisation was used with the Spearman correlation coefficients (rs), which were calculated for the inter-relationships among the studied elements. The results revealed associations of elements that are characterised by a similar geochemical behaviour in the sediments along the salinity gradient. Sedimentary Fe, Mg, Ti, Li, Co, Cs, Zn and V vary in relatively narrow ranges and tend to increase seaward. The Spearman correlation coefficients (rs) for the inter-relationships among these elements range from 0.8 to 0.95 (p ≤ 0.05 and n = 8). Sedimentary Mn also exhibits a significant positive correlation with Li, Co, V and Cs (rs = 0.8). Sedimentary As, Sn, Bi, U and Mo are generally uniformly low (rs range from 0.7 to 0.8) but tend to increase at stations 2 to 6 where resuspension processes at sediment disturbance events occur. The distribution of Ba, Sr and Ca is largely controlled by the total inorganic carbon (TIC) content in the sediments (rs range from 0.97 to 0.98). The strong positive correlation of Mn with Ca and TIC (rs = 0.85) indicates that Mn is largely associated with carbonates in the studied sediments. The distribution of Ni, Cr, Zr, Cu, Pb, Sb and particularly Ag was characterised by anomalously high concentrations in the estuarine part of the river–sea transect (sts. 3–4). The Spearman correlation coefficients (rs) for the inter-relationships among these elements range from 0.6 to 0.9.
SPECIATI ON OF MAJOR AND TRACE ELEMENTS IN SURFACE SEDIMENT
The total contents of the dithionite-soluble, oxalate-soluble, weak-acid-soluble and strong-acid-soluble forms of Fe, Mn, Li, Cr, Zn, Cu, Pb, Ni and Ag are provided in Table 3 and Figure 4. In this work, sodium dithionite served to mobilise both crystalline and amorphous Fe oxyhydroxides from the sediments [19]. Ammonium oxalate served to mobilise the easily soluble amorphous Fe-oxyhydroxides and acid-soluble fulvates [49, 50]. Acetic acid removed the labile metals in ion exchange positions, the easily soluble amorphous compounds of iron and manganese, the carbonates and the metals that are weakly held in organic matter [30]. Nitric acid removed the potentially mobilisable metals that are specifically sorbed onto clay particles, bound to easily soluble and resistant iron and manganese minerals or organic compounds, and integrated with discrete hydroxides, carbonates and sulphides [19, 21]. Additionally, the silicate-bound Fe was calculated as the difference between the total and dithionite-soluble forms and the crystalline Fe was determined as the difference between the dithionite-soluble and oxalate-soluble forms [50].
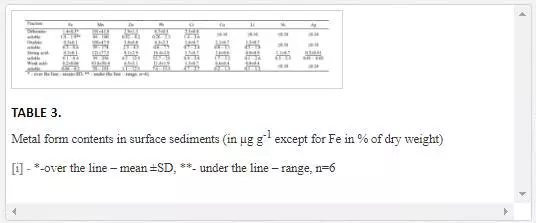
The total Fe content and the total and percent contents of its silicate-bound form (17–73% of the total content) increase, whereas the total and percent contents of the non-silicate-bound (27–83% of the total content) and crystalline forms (11–66% of the total content) decrease in the sediments along the salinity gradient. The total content of the amorphous form increases from the river to the sea, whereas its percent content varies insignificantly (14–17% of the total content) and is the highest in sediments in the riverine and frontal zone (sts. 1–2) and at the most seaward location (st. 8). The percent content of the weak-acid-soluble Fe, which is mostly comprised of easily soluble amorphous oxides, was constantly low along the salinity gradient (4–6% of the total content). The percent content of the strong-acid-soluble Fe increases from 7% to 13% of the total Fe and silicate-bound Fe contents. The total Mn content tends to increase in sediments along the salinity gradient. The total and percent contents of dithionite-soluble Mn (19–56%), oxalate-soluble Mn (19–61%), weak-acid-soluble Mn (18–42%) and strong-acid-soluble Mn (19–54%) are the highest in the coarsest sediments of the frontal zone (sts. 1–2). Seaward, the contents of the studied forms of Mn decrease in the sediments in the transitional sub-zone (sts. 3–4) and increase again in the marine end-member of the transect (st. 8). All extracts that were used appear to release comparably high amounts of Mn from the sediments (28–36% on average).
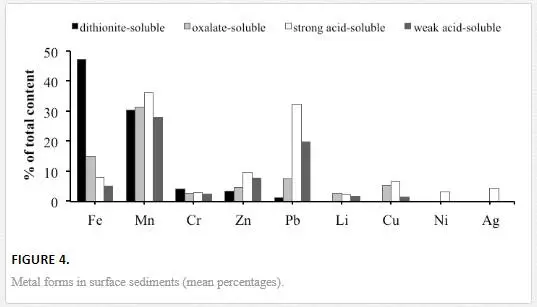
The contents of the dithionite-soluble form were negligible or below the detection limit for Li, Cu, Ni and Ag. This form comprises 2–12% (4.2% on average) of the total content for Cr, 1–4% (1.2%) for Pb and 0.5–6.5% (3.4%) for Zn (Figure 4). The contents of the oxalate-soluble form were negligible or below the detection limit for Ni and Ag. This form comprises 1–5% (2.6% on average) of the total content for Cr, 1–16% (7.5%) for Pb, 4–7% (4.5%) for Zn and 0.5–2% (1%) for Li. The contents of the weak-acid-soluble form were negligible or below the detection limit for Ni and Ag. This form comprises 1–4% (2.4 % on average) of the total content for Cr, 13–28% (19.8%) for Pb, 4–11% (7.7%) for Zn, and 0.4–2% (1.8%) for Li (Figure 4). The contents of the strong-acid-soluble form were negligible or below the detection limit for Ag. This form comprises 1–5% (2.6% on average) of the total content for Cr, 19–49% (32%) for Pb, 9–12% (9.5%) for Zn, 0.4–4% (2.1%) for Li and 1–7% (3.2%) for Ni. The percent contents of dithionite-soluble Pb, dithionite-soluble Zn, oxalate-soluble Pb, oxalate-soluble Zn, oxalate-soluble Cr, oxalate-soluble Cu and strong-acid-soluble Cu are the highest in the riverine and frontal zone (sts. 1–2) and generally decrease seaward with non-silicate Fe. The percent content of the strong-acid-soluble Pb is the highest in the riverine and marine sediments (sts. 1–2 and 8) and depleted in the transitional sub-zone at stations 3–4. The total and percent contents of the strong-acid-soluble Zn generally increase along the salinity gradient. The percent content of strong-acid-soluble Cr is uniformly low with a minimum in the sediment that is characterised by a maximum of total Cr (st. 4).
Figure 5 illustrates the distribution of the ecologically most significant weak-acid-soluble (labile) fraction along the river–sea transect. Mn and Pb have the highest percent contents of the labile form and exhibit similar spatial distributions. The sediments are enriched with labile Mn and Pb in the frontal zone and the marine end-part of the river–sea transect. This result contributes to the special affinity of Pb to Mn oxides in sediments. Percent contents of the labile forms of Fe and Zn are elevated in sediments that are enriched with crystallised Fe-oxides and detrital aluminosilicates (sts. 3–6). Cu and Cr exhibit the lowest contents of the labile form. The distribution of the labile Cu and Cr is complicated by a pronounced minimum in the sediment at station 4, which coincides with the maximum in the total Cu and Cr contents. Therefore, in the studied sediments, Cu and Cr are most likely bound to the residual mineral phase that is comprised of detrital heavy minerals (such as hornblende).
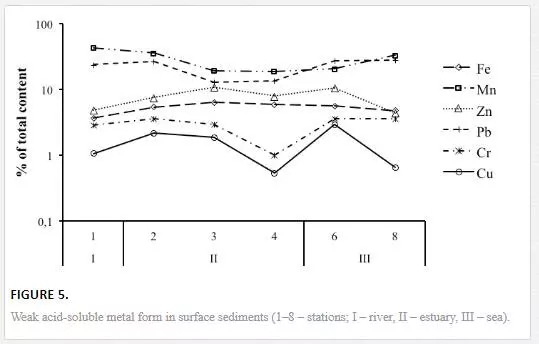
Discussion
ABUNDANCE AND DISTRIBUTION OF MAJOR AND TRACE ELEMENTS IN SURFACE SEDIMENTS
The hydrology of the riverine sub-zone of the studied part of the Cai River–Nha Trang Bay estuarine system is strongly influenced by the fill dam, which was constructed more than 15 years ago to prevent a saline water invasion into the Nha Trang city water supply system. The surface fresh-water layer flows seaward over the dam, while the upstream penetration of the near-bottom saline water lenses is blocked. The dam serves as a mechanical barrier that sharply decreases the river flow velocity and marks the frontal zone of the contact of fresh and saline waters. Seaward from the dam, the transitional waters are highly stratified with pronounced horizontal and vertical salinity gradients. In the marine sub-zone, the water-mass column becomes homogenized with a ‘calming down’ of river-flow currents.
The mean grain size of the sediments that were studied generally decreases from the river to the sea. The total content of detrital minerals (quartz, microcline, orthoclase and albite) generally decreases, whereas the total content of clay minerals (kaolinite, illite and clinochlore) and carbonates (calcite and aragonite) generally increases from the riverine to the transitional and bay sediments. This is due to the natural fractionation and deposition of the material of different grain sizes at sites determined by the hydrodynamic conditions of the water-mixing zone. The coarsest river material enriched in detrital minerals is deposited in the riverine part of the estuary at the sharp decrease of the river flow velocity enhanced by the dam. In the transitional waters at the near-bottom resuspension processes, the mixed-sized sediments enriched in silt and clay minerals are formed. In the marine part of the estuary, with a homogenization of the water column, most of the fine-grained material enriched in clay minerals and carbonates is deposited.
The observed distribution of major elements in the sediments illustrates the grain size and mineral fractionation processes. Si content is the highest in the riverine sediment and generally decreases seaward with the quartz content. Al, Fe, Ti, Mg and Mn (to a lesser extent) increase seaward in the sediments with the clay-sized materials. The strong positive correlation of Fe, Ti, Mg with Al and Li implies that the Fe-rich aluminosilicates (such as clinochlore and hornblende) are the dominant host minerals for Fe and the associated elements, although discrete oxide and detrital sulphide minerals may contribute to the accumulation of Fe and other elements at some sites. The abundance and distribution of Na and K are largely controlled by the Na- and K-aluminosilicate (such as albite, microcline and orthoclase) content in the sediments. The distribution of P is largely controlled by the abundance of minerals of the feldspar and amphibole groups (such as hornblende). The distribution of S is controlled to a greater extent by the abundance of sulphates (such as gypsum) and detrital and/or authigenic sulphides. The strongest positive correlation of TIC with Ca implies that carbonates (such as aragonite and calcite) are the dominant host minerals for these elements. TOC content is relatively low and shows no significant correlation with the studied major and trace elements in the sediments along the river–sea transect. This may be due to the intensive microbial decomposition of particulate organic matter, which occurs in the water column during estuarine sedimentation processes [40, 41]. The post-depositional diagenetic reactions, which are enhanced by resuspension processes at sediment disturbance events (such as tides, storms and upwelling), may also contribute to a destruction of sedimentary organic matter and the formation of organic-poor sediments [9, 23, 39]. The organic geochemistry and sources of organic matter in the sediments of the Nha Trang Bay and Cai River were studied by V.I. Peresypkin and N.V. Lobus. The observed TOC and n-alkanes distribution showed that sediments from the studied basin tend to be planktonogenic-terrigenous with major input of organic matter from terrestrial plant remains. The presence of petroleum hydrocarbons in some samples in the Nha Trang Bay indicated the input of petroleum products from industrial wastes [26, 38].
The distribution of trace elements in sediments is strongly influenced by the water-column stratification because of the natural fractionation and deposition of materials of different grain sizes at sites which are determined by hydrodynamic conditions [16, 46]. In estuaries, the flocculation and coagulation of riverine microcolloids are initiated when the salinity increases. These processes are accompanied by a rapid scavenging of dissolved trace elements from the water column. Further deposition of newly formed aggregates contributes to the enrichment of sediments with trace elements [33, 43]. To normalise the obtained geochemical data for the grain-size effects and identify the enrichment zones along the salinity gradient, the metal/Al ratios were calculated (Figure 3) [11, 30]. The observed distribution of the normalized trace element contents reflects the association with and/or inclusion of Fe, Mg, Ti, Li, Co, Cs, Zn, V and Mn (to a lesser extent) in the lattices of ferromagnesium silicates (such as hornblende) and clay minerals (such as kaolinite, illite and clinochlore), which constitute the bulk of the fine-grained sedimentary material. These elements are most likely controlled by the accumulation of their most fine-grained aluminosilicate host minerals and materials in the sea floor depression of the marine sub-zone [29]. As, Sn, Bi, U and Mo tend to increase in the transitional and marine sub-zones at stations 2 to 6 where resuspension processes at sediment disturbance events occur. An earlier study showed that Fe/Mn-oxides control the immobilisation of Mo, As and U in the solid phase in modern turbidities [7]. The kinetics of the particle concentration effect (PCE) is likely higher near the estuarine turbidity maximum (ETM), where colloidal-bound trace metals coagulate over shorter periods [3]. Ba, Sr and Ca distribution is largely controlled by the total inorganic carbon (TIC) content in the sediments. These elements form low-soluble carbonates in aquatic environments [44]. The strong positive correlation of Mn with Ca and TIC (rs = 0.85) indicates that Mn is largely associated with carbonates in the studied sediments. The distribution of Ni, Cr, Zr, Cu, Pb, Sb and particularly Ag was characterised by anomalously high concentrations in the transitional part of the river–sea transect (sts. 3–4). The local sediment enrichment in Ni, Cr, Zr, Cu, Pb, Sb and Ag might occur because of a point of anthropogenic contamination from the harbour, domestic and industrial wastes. Thus, metal-based antifouling systems may play a major role in the trace heavy-metal contamination at the harbour [4]. The enhanced content of detrital heavy minerals may also result in anomalous natural metal concentrations [18]. Thus, the Zr concentration in the sediments is most likely heightened because of the enhanced content of Zr-rich minerals such as zircon [45]. To estimate the extent of heavy-metal contamination in the sediments, it was necessary to establish the natural background metal levels, separate the background levels from the anthropogenic inputs and account for the natural variability in the composition of the sediments [29, 32].
There are few published literature data on the total metal levels in coastal sediments from Central Vietnam and Khanh Hoa province in particular. Our estimates of Li, Mn, Ni, V, Pb, Cr, Zn As, Cd, U and Hg are higher or correspond to the measured metal levels in sediments from the coastal lagoons Thuy Trieu and Dam Nai [42]. The reported Hg levels in the present study correspond to the data of N.V. Lobus, which revealed the negative geochemical anomaly of Hg in Central and Southern Vietnam [25]. Due to the lack of available data on metal concentrations of regional sediments, the Clark values for texturally equivalent reference sediment materials (shale, pelagic clays and world river bed sediments) were used as a measure of natural background levels [30]. Comparing to the reference Clark concentrations, the measured levels of U, Bi and especially Ag in the sediments are heightened. U and Bi contents are relatively constant along the river–sea transect and exhibit a negligible elevation in the turbidity zone. Presumably, these disparities were related to regional differences in sediment chemistries rather than to any enhanced contamination. On the contrary, Ag content significantly exceeds the reference values in most of the studied samples and exhibits a sharp maximum in the transitional sub-zone (sts. 3–4). The results of the comparative study of trace element contents and SQGs also indicate that among the studied elements, Ag may contribute to the toxicity of surface sediments [27]. Because element speciation contributes to toxicity and bioavailability in natural systems, the comparative analysis of ecologically significant forms of the studied elements was essential [9, 10].
SPECIATION OF MAJOR AND TRACE ELEMENTS IN SURFACE SEDIMENTS
Major and trace elements are bound to a variety of sediment fractions that range from easily extractable (and bioavailable) to resistant residual mineral phases [13, 48]. According to the comparative extractability from sediments, Ag, Ni, Li, Cr and Cu are low-labile and mainly occur in the residual phase. These metals were mainly extracted in the detrital fraction, which emphasises the importance of natural weathering and erosion in drainage basins. Therefore, the previously obtained anomalous concentrations of Ag in the sediments of the Cai River estuary and Nha Trang Bay are most likely related to the enhanced content of metal-rich detrital heavy minerals such as Ag-sulphosalts of the freieslelebenite and pyrargyrite groups that may originate from the detrital sulphide minerals of regional bedrocks. The pronounced enrichment of sediments in detrital Ag, Ni, Cu and Cr in the transitional sub-zone (sts. 3–4) may reflect the small-scale fractionation processes such as local deposition of material of particular grain size and mineralogical composition. Fe and Zn are moderately labile and occur in the less resistant phases such as crystallised Fe/Mn oxides and organic compounds that may be a threat in the long term. Mn and Pb are labile, held in ion exchange positions, bound to easily soluble amorphous Fe/Mn compounds and weakly held in organic matter. This result supports the special affinity of Pb to Mn oxides in soils and sediments [9]. The high levels of acid-soluble Pb (28–32% of the total content) compared to previously studied estuarine and coastal sediments suggest a contamination problem in the Nha Trang Bay, which arises from the Cai River discharges, while the elevated level of easily reducible Pb fraction (7.5% of the total content) also contributes to the anthropogenic input of Pb [18, 20]. The sources of the elevated contents of detrital Ag and labile Pb in the studied sediments need further study.
The speciation of Fe and Mn illustrates the natural fractionation processes in the estuarine system that was studied. Fe in the soils of the Cai River catchment area is largely present as non-silicates, which account for 85–97% of the total Fe content, and the crystalline forms are dominant (90% of the total Fe content) [34]. Sedimentation of the rich in non-silicates riverine material and flocculation of the newly formed amorphous Fe oxyhydroxides lead to the accumulation of Fe in the sediments in non-silicate form in the riverine and frontal zone (sts. 1–2) of the estuary. In the bay, most of the fine-grained clay material that largely comprises Fe-rich aluminosilicates is deposited (st. 8). The most bioavailable parts of Mn are concentrated in coarse riverine sediments (in the form of individual Mn oxides) (st. 1) and fine-grained marine sediments (in the form of easily soluble amorphous Mn-containing Fe-oxyhydroxides and carbonates) (st. 8). The speciation of trace elements revealed that riverine and frontal zone sediments (sts. 1–2) are rich in actual and potential bioavailable forms of Mn, Pb, Cr and Cu because of scavenging and co-precipitation by Fe/Mn oxyhydroxides and organic microcolloids. Marine sediments (sts. 6–8) are rich in bioavailable forms of Mn and Pb because of the association with amorphous Fe-oxyhydroxides and carbonates. The transitional sediments are depleted in bioavailable forms in most of the studied metals.
The contents of oxalate-soluble (amorphous) forms were higher than the contents of dithionite-soluble (non-silicate) forms at all sites for Pb and at most sites for Cu and Zn. Therefore, the most bioavailable parts of Pb, Cu and Zn are bound to amorphous Fe and Mn oxyhydroxides and acid-soluble organic compounds. Higher percentages of Cr are obtained in the more resistant reducible (dithionite-soluble non-silicate) fraction, which indicates the inclusion of Cr in crystalline iron compounds. The contents of the strong-acid-soluble forms significantly exceed those of the weak-acid-soluble forms at most sites for Pb and, to a lesser extent, for Cu and Zn. Therefore, the potentially mobilisable Cu, Zn and Pb may be bound to clay minerals and to resistant organic compounds. Assuming that the mean determined amounts of the strong-acid-soluble and weak-acid-soluble forms are a measure of the potential metal bioavailability in sediments, the studied elements can be arranged in the following increasing order of average potential bioavailability: Ag<Ni<Co<Li<Cu<Cr<<Fe<Zn<<Pb<Mn (Figures 4 and 5). This sequence is true for sediments in different sub-zones of the water-mixing zone: river, estuary (transitional waters) and sea (bay).
The most bioavailable parts of the studied trace metals are associated with easily soluble amorphous Fe and Mn oxyhydroxides. This result supports the fact that Fe and Mn oxyhydroxides are readily complex trace metals and control the bioavailability in sediments [3, 6, 43]. With the widespread occurrence of Fe and Mn oxyhydroxides and because macrofauna primarily reside in aerobic environments, the role of oxyhydroxides in controlling metal bioavailability is tremendously important and requires further investigation.
Conclusions
The major-element composition of the sediments from the Cai River estuary and Nha Trang Bay generally corresponds to the reference background values. The Si content tends to decrease, whereas the contents of Al, Fe, Ti, Mn, Mg, Na, K, S and P increase from the riverine to marine sediments because of a general grain size decrease.According to the sediment quality guidelines (ERL/ERM) and the reference background values, most of the studied trace elements were below the threshold levels, whereas the total content of Ag significantly exceeded the natural levels. Along the salinity gradient, several zones of metal enrichment occur in the surface sediments because of the geochemical fractionation of the riverine material. Fe, Mn, Mg, Ti, Na, K, Li, Co, Cs, Zn and V are largely controlled by the accumulation of their most fine-grained aluminosilicate host minerals at the sites which are determined by hydrodynamic conditions. As, Sn, Bi, U, Cd and Mo are most likely controlled by the co-precipitation with the dissolved and particulate materials of the river discharge in the transitional waters under turbidity processes. Ca, Ba and Sr are largely controlled by the carbonate abundance in the sediments. Ni, Cr, Zr, Cu, Sb and particularly Ag show a pronounced enrichment at the sites, which is most likely determined by the local accumulation of metal-rich detrital heavy minerals.
The combined method of single-chemical-reagent extractions revealed labile to relatively stable associations of metals in the surface sediments. The studied elements can be arranged in the following increasing order of bioavailability: Ag<Ni<Co<Li<Cu<Cr<<Fe<Zn<<Pb<Mn. The low-labile Ag, Ni, Co, Li, Cr and Cu mainly occur in the residual phase. The severe enrichment of Ag most likely originates from metal-rich detrital heavy minerals such as Ag-sulphosalts. The moderately labile Fe and Zn are largely associated with crystallised Fe/Mn oxides and resistant organic compounds. The labile Mn and Pb are mainly concentrated with easily soluble amorphous Fe/Mn compounds and carbonates. The elevated levels of acid-soluble and easily reducible forms of Pb (up to 36% of the total content) may indicate an anthropogenic origin of Pb in the estuarine system, which requires special study. In the river–sea transect, the riverine- and frontal zone sediments are mostly rich in bioavailable forms of Mn, Pb, Cr and Cu, whereas the marine sediments are rich in bioavailable forms of Mn and Pb.Mineralogy, grain size and depositional conditions generally determine the abundance and distribution of elements in the studied sediments. Natural (such as turbidities) and human-generated (such as urban and industrial activities) pressures affect the abundance and speciation of potential contaminants (such as trace metals) and may change their bioavailability in the studied estuarine system. Overall, iron and manganese oxyhydroxides largely control the trace-metal bioavailability in the sediments.